nasa mars experiment的問題,透過圖書和論文來找解法和答案更準確安心。 我們找到下列問答集和資訊懶人包
nasa mars experiment的問題,我們搜遍了碩博士論文和台灣出版的書籍,推薦Von Ehrenfried, Manfred "Dutch"寫的 The Artemis Lunar Program: Returning People to the Moon 和的 Advances in Remote Sensing and Geo Informatics Applications: Proceedings of the 1st Springer Conference of the Arabian Journal o都 可以從中找到所需的評價。
另外網站Mars Oxygen In-Situ Resource Utilization Experiment (MOXIE)也說明:NASA is preparing for human exploration of Mars, and MOXIE will demonstrate a way that future explorers might produce oxygen from the Martian atmosphere for ...
這兩本書分別來自 和所出版 。
長庚大學 醫學影像暨放射科學系 趙自強、董傳中、李宗其所指導 江悅的 應用於相對生物效應及微電子可靠度測試的輻射品質評估方法 (2020),提出nasa mars experiment關鍵因素是什麼,來自於微劑量學、相對生物效應、輻射可靠度、蒙地卡羅模擬。
而第二篇論文國立高雄師範大學 地理學系 何立德所指導 吳梅蘭的 恆春半島風吹沙晚全新世攀爬沙丘之地形演育研究 (2020),提出因為有 攀爬沙丘、粒徑分析、光螢光定年、風吹沙、恆春半島的重點而找出了 nasa mars experiment的解答。
最後網站Science Investigations - NASA's Mars Exploration Program則補充:This information is used to determine the rover's condition and assess results of science experiments. The rover¹s UHF antenna is also used for the return ...
The Artemis Lunar Program: Returning People to the Moon
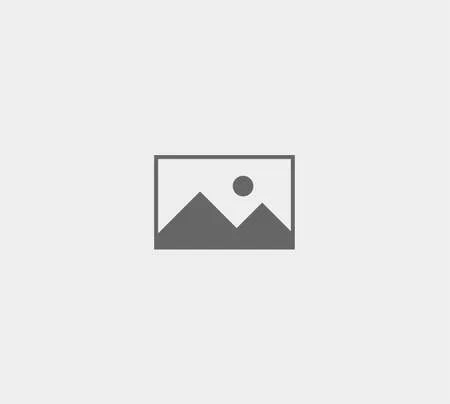
為了解決nasa mars experiment 的問題,作者Von Ehrenfried, Manfred "Dutch" 這樣論述:
NASA has made a firm decision to go back to the Moon in the very near term, which will provide us with many valuable lessons for any future trips to Mars. This "Lunar Gateway" approach--and in particular, the new Artemis program--will define space missions for the next decade and beyond.This book pr
ovides the first comprehensive overview of the upcoming Artemis program, which is planning the first human flights back to the Moon. Dutch von Ehrenfried covers all aspects of the program, including the specific modules, vehicles, lander, payloads and experiments being planned. The role of governmen
t and commercial entities will be discussed, along with how Artemis is expected to influence future missions to Mars."Moon to Mars" has become the theme of the U. S. space exploration. This book helps readers understand why this is, and what they can expect to see from the Artemis program in the nex
t few years. Dutch Von Ehrenfried has worked in both the space flight and aviation fields for about 25 years. He was a NASA Flight Controller in Mission Control for many Mercury, Gemini and Apollo Missions. Dutch also worked on some of the Apollo Lunar Experiment Packages from an operations point
of view as well as experiments that went into Skylab from an Earth Resources Program perspective. He later worked in the NASA Headquarters Space Station Task Force and the Program Office as a contractor for about 10 years, and the FAA Aviation Safety Office for a year. In recent years he has written
several insightful books in the Springer-Praxis series.
應用於相對生物效應及微電子可靠度測試的輻射品質評估方法
為了解決nasa mars experiment 的問題,作者江悅 這樣論述:
Table of Contents摘要 iiiAbstract ivChapter 1. Introduction 1Chapter 2. Radiation Environments and Their Quality 72.1. RADIATION QUANTITY AND QUALITY 72.2. RADIATION ENVIRONMENT IN THIS STUDY 92.2.1. Radiation for semiconductor industrial practice 102.2.2. Radia
tion for medical practice 122.3. SUMMARY 20Chapter 3. Microdosimetry and its simulation and measurement 213.1. CONCEPTS OF MICRODOSIMETRY 243.2. MONTE CARLO SIMULATION 353.3. MICRODOSIMETRY MEASUREMENT 403.4. SUMMARY 46Chapter 4. Lineal energy of proton in s
ilicon 474.1. THE DIFFERENCE BETWEEN LINEAL ENERGY AND LET 474.2. MICRODOSIMETRY SIMULATION 534.3. RESULTS AND DISCUSSIONS 574.3.1. Effect of SV thickness on y distribution 574.3.2. Lineal energy contribution from various secondary species 634.3.3. Effect of vario
us physics models on secondary yields 694.4. SUMMARY 70Chapter 5. Equivalence of Neutrons and Protons in Single Event Effects Testing 725.1. SINGLE EVENT EFFECT TESTING – METHODS AND FACILITIES 725.2. PROCESS FOR EQUIVALENCE VALIDATION 755.2.1. Monte Carlo Simulation
775.2.2. Material Structure 795.2.3. Data Analysis 815.3. RESULTS AND DISCUSSIONS 825.3.1. LET Difference between Neutrons and Protons 825.3.2. Secondary Particle Yield Difference between Neutronand Proton 885.3.3. LET Difference between Layer Structures with andwit
hout SiGe 915.3.4. Secondary Particle Yields Difference between Layer Structure with and without SiGe 935.3.5. Energy Deposition Difference between Neutronsand Protons 955.4. SUMMARY 99Chapter 6. Silicon equivalent gas in silicon equivalent proportional counter 1016.1.
SILICON EQUIVALENT GAS 1016.2. SIMULATION AND ANALYZATION METHODS FOR SE GAS SELECTION 1036.3. RESULTS AND DISCUSSION 1046.3.1. LET spectra 1046.3.2. Secondary particle yields 1056.4. SUMMARY 112Chapter 7. High Z material enhanced RBE 1147.1. RADIATION SENSI
TIZERS IN RADIATION THERAPY 1147.2. RBE SIMULATION AND CALCULATION METHODS 1177.2.1. MKM simulation 1177.2.2. DSB simulation 1207.3. RESULTS AND DISCUSSION 1217.3.1. Verification for microdosimetry simulation 1217.3.2. Microdosimetry spectra and RBE 1237.3.3.
Secondary electron spectra 1307.3.4. Correlation of DSB with electron energy 1327.3.5. Spectra of DSB 1337.4. SUMMARY 134Chapter 8. Conclusion 136References 138 List of FiguresFigure 1 1 LET threshold of SEEs vs. Feature size [6] 4Figure 1 2 (a) mechanism of total
ionization effect, (b) ΔVtm vs. time diagram due to TID [4] 4Figure 1 3 (a) Ionizing radiation generates charge, (b) Negative charge moves to the positive electrode to generate current, (c) Potential difference generates current, and (d) Current vs. time diagram due to a single event under revers
e bias[7] 5Figure 2 1 Example of a CMOS structure and mechanism of single event effect. (A) is the event from the heavy ions. (B) from the natural particle or proton. 12Figure 2 2 Schematic comparison of the local dose distributions (left) and corresponding spatial DSB distributions (right) fo
r low energetic (top) and high energetic (bottom) carbon ions. Assumed DSB yields are 50 DSB and 0.5 DSB for the low energetic and high energetic ions, respectively [33] 18Figure 2 3 Representation of a 10mGy dose delivered from gamma 60Co (left) and the same dose delivered by 1 MeV neutrons (rig
ht) in a cell volume of 150 cell of 5 µm diameter [37] 19Figure 2 4 The explanation of domain in microdosimetry kinetic model 19Figure 3 1 Specific energy (dE/dm) deposited by radiation in matter as a function of mass with the macroscopic dose being constant. 23Figure 3 2 lineal energy dist
ribution of tissue irradiated by 250 kVp X-ray. Linear scale. 31Figure 3 3 lineal energy distribution of tissue irradiated by 250 kVp X-ray. Log scale. 32Figure 3 4 lineal energy distribution of tissue irradiated by 250 kVp X-ray. Semi-log scale. 33Figure 3 5 dose weighted lineal energy dis
tribution of tissue irradiated by 250 kVp X-ray. Semi-log scale. 34Figure 3 6 Lineal energy spectra of different sensitive volumes in silicon irradiated by a 200 MeV proton beam. 35Figure 3 7 Block diagram of basic process of Monte Carlo method in radiation transportation code 39Figure 3 8
A sketch of the cross-sectional view of SEPC with its component 43Figure 3 9 block diagram of the SEPC measurement system 43Figure 3 10 Simulated lineal energy spectra for SEPC irradiated by 50 kVp and 150 kVp X-ray 45Figure 4 1 The geometry setup in this study. The silicon is with natural
isotope abudence, density is 2,330 mg/cm3 and mean excitation potential I = 173 eV. 57Figure 4 2 Lineal energy spectra of different sensitive volumes in silicon irradiated by a 200 MeV proton beam. 61Figure 4 3 Cumulative distribution function of kinetic energy of secondary particles generate
d by 200 protons irradiated on silicon 62Figure 4 4 Lineal energy spectra of different sensitive volumes in silicon irradiated by a 200 MeV proton beam (log y scale). 63Figure 4 5 y spectra in 100 nm silicon irradiated by a 200 MeV proton beam 67Figure 4 6 Secondary particle yields in 100 n
m silicon irradiated by a 200 MeV proton beam using various physics models. BIC represents the Binary cascade model. BERT represents Bertini cascade model. HP represents high precision add-on 70Figure 5 1 The Los Alamos Neutron Science Centre (LANSCE) broad band neutron spectrum used in this stud
y [112]. 76Figure 5 2 The layer structure (a) without SiGe and (b) with SiGe used in this simulation (not to scale). 80Figure 5 3 Linear energy transfer (LET) spectra in a structure without silicon-germanium (SiGe) irradiated by 63, 105, 150, 200, and 230 MeV proton and LANSCE neutron. 84Fi
gure 5 4 The LET contribution from He, Mg, and Al generated by the 200 MeV proton and the LANSCE neutron. In parentheses, the first symbol represents incident particles, and the second symbol represents particles that contribute to the LET. 85Figure 5 5 LET spectra in a structure without SiGe fro
m 10, 30, 50, 63, and 200 MeV protons and LANSCE neutron. 86Figure 5 6 The secondary particle yields in structure without SiGe irradiated by 63, 105, 150, 200, and 230 MeV protons and LANSCE neutron. 89Figure 5 7 LET spectra of the structure with and without SiGe irradiated by 63 and 230 MeV p
rotons and LANSCE neutron. The plot is in log-log scale. 92Figure 5 8 The secondary particle yields in the structure with and without SiGe irradiated by 63 and 230 MeV protons and LANSCE neutron. 94Figure 6 1 Simulated LET spectra in the SEPC cavity for proton irradiations of (a) 63 MeV and (b
) 230 MeV. Results of cavity gas Si, CCl4, propane, Ne and Ar are plotted. 107Figure 6 2 Simulated LET spectra in the SEPC cavity for neutron irradiations of (a) 4.44 MeV and (b) 750 MeV. Results of cavity gas Si, CCl4, propane, Ne and Ar are plotted. 108Figure 6 3 Evaluation index, EI, of LET
spectra for different SEPC cavity gases under proton and neutron irradiations 109Figure 6 4 Simulated secondary particle yields in the SEPC cavity for proton irradiations of (a) 63 MeV and (b) 230 MeV. Results of cavity gas Air, Ar, CCl4, CO2, He, Kr, Ne, propane, Si and Xe are plotted 110Fig
ure 6 5 Simulated secondary particle yields in the SEPC cavity for neutron irradiations of (a) 4.44 MeV and (b) 750 MeV. Results of cavity gas Air, Ar, CCl4, CO2, He, Kr, Ne, propane, Si and Xe are plotted. 111Figure 6 6 Evaluation index, EI, of secondary particle yields for different SEPC cavity
gases under proton and neutron irradiations 112Figure 7 1 Input spectra for Monte Carlo simulation. The spectra are measured by INER and modified for Geant4 GPS input format. 119Figure 7 2 Comparison of simulation data with measurement data. Dots represent the simulation data with 20 points p
er decade. The continuous line shows the measurement data in INER’s medium energy X-ray air kerma rate calibration system. 123Figure 7 3 Microdosimetry spectra of 80 kVp La transmission X-ray w/ and w/o iodine 125Figure 7 4 Microdosimetry spectra of 250 kVp X-ray w/ and w/o iodine 126Figure
7 5 Secondary electron spectra. (A) The secondary electron of 80 kVp La Fluorescence X-ray. (B) The secondary electron of 250 kVp X-ray 131Figure 7 6 The yields of DSB for different electron. The energy step is set 20 energies per decade in log scale in simulation. The cubic spline method is app
lied to do the interpolation. The fitting curve is shown in 10 eV per step. 133Figure 7 7 DSB yield. The DSB yield is the product of secondary electron and DSB cross-section. 134 List of TablesTable 4 1 The calculated LET using mean energy of secondary particles generated by 200 MeV proton irr
adiate on silicon 68Table 5 1 Evaluation index (EI) for LET in layer structure without SiGe. 88Table 5 2 EI for secondary particle yields in layer structure without SiGe. 90Table 5 3 EI for LET in layer structure with SiGe. 94Table 5 4 EI for secondary particle yields in layer structure
with SiGe. 95Table 5 5 Energy deposition analysis results for the layer structure without SiGe for 1010 neutron/proton incident 97Table 5 6 Energy deposition analysis results for the layer structure with SiGe for 1010 neutron/proton incident 98Table 7 1 Frequency mean lineal energy, dose me
an lineal energy and calculated RBE for each irradiation condition 127Table 7 2 Relative dose in cavity and wall for each irradiation condition in same fluence 128Table 7 3 Relative number of secondary electrons generated by unit dose and overall RBE 129
Advances in Remote Sensing and Geo Informatics Applications: Proceedings of the 1st Springer Conference of the Arabian Journal o
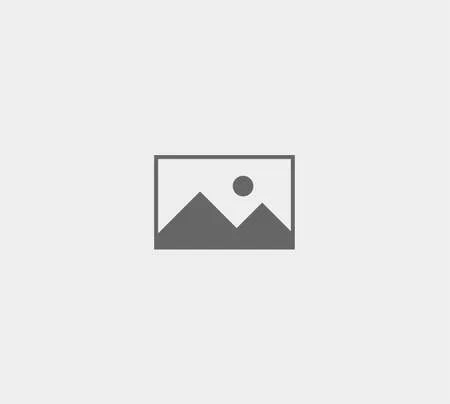
為了解決nasa mars experiment 的問題,作者 這樣論述:
Prof. El-Askary received his Ph.D. in Computational Sciences and Informatics from George Mason University in 2004. He is the 2015 recipient of the Chapman University’s elite Senior Wang-Fradkin Professorship award. In 2016, he was named as the regional coordinator on a $3 million Euro grant from the
European Union’s (EU) Horizon 2020. The three year project, known as GEO-CRADLE, deals with Coordinating and integrating state-of-the-art Earth Observation Activities in the regions of North Africa, Middle East and Balkans and Developing Links with GEO related initiatives toward GEOSS. Through this
work he with the research team were able to deliver the first analytical solar Atlas of Egypt that is now considered to be the official document of the government for solar investment. This work was recently presented at Planet Earth Institute at the Royal Society in London during a seminar that di
scussed the future of solar energy in Africa. His research interests include dust storms monitoring and detection using different remote sensing technologies as well as studying other extreme events. He is also involved in studying air pollution problems over mega cities due to natural and man-made
effects as well as climate change and its impacts on sea level rise and coral reefs for coastal areas. His research also included using earth observations in studying impact of sever dust storms anomalous chlorophyll outbreaks in the marine environment, hurricanes intensification as well as transpor
t of microbes’ causing Kawasaki disease outbreaks. Recently Prof. El-Askary has been focusing on using earth observations for water resources management, precision agriculture along the sustainable development goals. Today Prof. El-Askary views himself as an Earth System Scientist with a major inter
est in natural hazards, atmospheric events and using renewable energy as the only way to address global climate change issues. Prof. El-Askary has published over a 100 refereed research publications, conferences full paper and book chapters in these research areas. Dr. El-Askary’s research has been
supported by National Science Foundation, NASA, United States Department of Agriculture and European Union. Dr. El-Askary has received the Saudi Arabia award hosted by the Arab Administrative Development Organization (ARADO) affiliated with the League of Arab states for the best published article in
Environmental Management among 150 articles in 2006. He is also member of the Institute of Electrical and Electronics Engineers (IEEE), AGU, EGU, COSPAR, and Phi Beta Delta Honor Society. Dr. Essam Heggy is a Research Scientist at the Microwave Systems, Sensors and Imaging Lab (MiXIL), at the Vite
rbi School of Engineering at the University of Southern California and affiliate of the Rosetta project at the NASA Jet Propulsion Laboratory. Heggy obtained both his MSc. and Ph.D. respectively in 1999 and 2002 with distinguished honors from the Paris VI University in France (UPMC-Sorbonne). His re
search focuses on understanding water evolution in Earth’s arid environments as well as planetary surfaces using radar surface and subsurface characterization methods. His research particularly focuses on understanding volatile evolution in the North African Sahara and Arabian Peninsula, as well as
Mars, the Moon, Jovian Icy satellites and Near-Earth Objects. His work involves probing structural, hydrological and volcanic elements in terrestrial and planetary environments using different types of radar imaging and sounding techniques as well as measuring the electromagnetic properties of rocks
in the radar frequency range. He is currently a member of the science team of the MARSIS instrument aboard the Mars Express orbiter (2003-present), the Mini-SAR experiment aboard Chandrayaan-1, the Mini-RF experiment on board the Lunar Reconnaissance Orbiter (2008-present), the CONSERT radar experi
ment aboard the Rosetta mission (2004-present) and the WISDOM GPR onboard ExoMars 2020 Rover (2008 to Present). He also edited a special JGR-Planets (AGU) volume on terrestrial and planetary radars. He is on the founding editorial board of the Journal of Arctic Geoscience ARKTOS (Springer), Geoscien
ces (MDPI), National Geographic and co-chaired several sessions in international conferences and workshops on terrestrial and planetary radar subsurface imaging including AGU, LPSC and IEEE meetings. Prof. Pradhan received the B.Sc. degree with honors from Berhampur University (India), the M.Sc. deg
ree from the Indian Institute of Technology (IIT) in Bombay (India), and the M.Tech. degree in Civil Engineering from the IIT in Kanpur (India) and Dresden University of Technology (Germany). He received the Ph.D. degree in GIS and Geomatics Engineering from the University Putra Malaysia. From 2008
to 2010 he was a recipient of the Alexander von Humboldt Research Fellowship from Germany. In 2011, he received his Habilitation in Remote Sensing from Dresden University of Technology (Germany). Since March 2015, he is serving as the Humboldt Ambassador Scientist for the Alexander Von Humboldt Foun
dation (Germany). Dr. Pradhan is also the recipient of the prestigious German Academic Exchange Research (DAAD) Fellowship Award, Saxony State Fellowship from 1999 to 2002, Keith Atherton Research Award, and Georg Forster Research Award from German Government. He is currently Faculty Member of Dept.
of Civil Engineering, University Putra Malaysia. He has more than sixteen years of teaching, research, consultancy and industrial experience. Out of his more than 450 articles, more than 276 have been published in science citation index (SCI/SCIE) technical journals. He has written two books in GIS
data compression and disaster management and edited three volumes, and written 12 book chapters. He has recently submitted two new books for publication with Springer. He specializes in Remote Sensing, GIS application, and soft computing techniques in natural hazard and environmental problems. His
published work has been widely cited by his peers with more than 4800 citations in SCOPUS database allowing him to reach a h-index of 47. He has completed 20 research projects. Most recently, he has been selected as a 2016 Web of Science High Cited Researcher. Dr. Pradhan is a member of many profess
ional bodies such as Committee of Space Research (COSPAR), Senior Member of IEEE, United Nations Outer Space Research Programme (UNOOSA) and many more. He sits as a board member of many national programs in Malaysia and South-East Asia. He is a regular reviewer for many international bodies alike Du
tch Research Council, European Science Foundation, Austrian Science Foundation, Research Council UK (RCUK) and many more. He has been also active with teaching and supervising of many Ph.D., MSc. and undergraduate students. Dr. Pradhan has widely travelled abroad visiting more than 55 countries to p
resent his research findings (e.g. Germany, USA, Netherlands, UK, Switzerland, Turkey, South Korea, Japan, Indonesia, Thailand and many more). In 2013 Dr. Pradhan joined the AJGS as an Associate Editor responsible for evaluating submissions in the fields of Environmental, Geo-Informatics and Geotech
nical Sciences. Dr. Lee completed his B.Sc. in Geology (1991), a M.Sc. in GIS-based geological hazard mapping (1993) and a Ph.D. in landslide susceptibility mapping using GIS (2000) at Yonsei University in Seoul (Korea). He is currently a Principal Researcher at the Geological Research Division of K
orea Institute of Geoscience and Mineral Resources (KIGAM). He is also a Professor at the University of Science and Technology (UST) in Daejeon (South Korea). He started his professional career in 1995 as a researcher at KIGAM. He spent many years as a part-time lecturer in many universities. He car
ried out many international cooperative research projects in the fields of mineral potential and geological hazard in Brazil, Bhutan, Cambodia, China, Indonesia, Malaysia, Philippines, Thailand and Vietnam. He also managed several times the Korea International Cooperation Agency (KOICA) Internationa
l Training Program and gave lectures in the fields of Mineral Exploration and GIS/RS for participants from many countries. His research interest includes geospatial predictive mapping with GIS and RS such as landslide susceptibility, ground subsidence hazard, groundwater potential, mineral potential
and habitat mapping. He has co-authored more than 100 research articles in refereed journals and is an ISI highly cited researcher (ca. 5000 citations, h-index 37). In 2015 Dr. Lee joined the AJGS as an Associate Editor responsible for evaluating submissions in Environmental, Geotechnical and Appli
ed Sciences.
恆春半島風吹沙晚全新世攀爬沙丘之地形演育研究
為了解決nasa mars experiment 的問題,作者吳梅蘭 這樣論述:
臺灣四面環海,海岸沙丘多發育於河口附近或地勢平緩的海岸地區,是海岸沉積物運動體系的一環,為陸地、海洋和大氣系統三者交互作用的結果 (Nordstrom et al., 1990)。恆春半島的風吹沙景觀是臺灣著名的海岸沙丘地景之一,目前僅有Ho et al. (2017) 對風吹沙的崖頂沙丘 (cliff-top dune) 進行研究,根據風吹沙崖頂沙丘形成時空背景提出地形演育模式。位於風吹沙海崖底部的攀爬沙丘 (climbing dune,又稱爬升沙丘),是海灘和崖頂沙丘之間重要的連結,然而尚未有人對其進行詳細的研究。本研究調查風吹沙攀爬沙丘的地形與沉積層特徵,採集風積物至實驗室內進行粒徑分
析,並使用碳十四定年法與光螢光定年法建立風沙堆積的時序,藉此重建攀爬沙丘的發育歷史。研究結果顯示形成於全新世晚期的攀爬沙丘,主要歷經了三次沙丘堆積時期,明顯受到古氣候的控制。風成沉積物中的膠結硬層指示了風沙堆積停止後的沙丘古地形面,這些古地形面的坡度可能反映了風沙堆積時的風力強弱,而風力強弱的變化也反應在風積物的粒徑變化與碳十四年代資料上。攀爬沙丘與崖頂沙丘的風沙堆積歷史大致可以對比,但細部變化並非全然一致,顯示地形與地表作用之間的回饋影響。
想知道nasa mars experiment更多一定要看下面主題
nasa mars experiment的網路口碑排行榜
-
#1.Perseverance, NASA's newest Mars rover - The Planetary ...
NASA's Perseverance rover will search for past life on Mars and store samples ... means we could run the same science experiments in multiple laboratories. 於 www.planetary.org -
#2.7 Things to Know About the NASA Perseverance Rover About ...
NASA Mars 2020 Perseverance Rover Spacecraft Approaches Mars ... RIMFAX (short for Radar Imager for Mars' Subsurface Experiment) will use ... 於 scitechdaily.com -
#3.Mars Oxygen In-Situ Resource Utilization Experiment (MOXIE)
NASA is preparing for human exploration of Mars, and MOXIE will demonstrate a way that future explorers might produce oxygen from the Martian atmosphere for ... 於 mars.nasa.gov -
#4.Science Investigations - NASA's Mars Exploration Program
This information is used to determine the rover's condition and assess results of science experiments. The rover¹s UHF antenna is also used for the return ... 於 mars.nasa.gov -
#5.NASA just found these organic molecules on Mars for the first ...
Abstract: The wet chemistry experiments on the Sample Analysis at Mars instrument on NASA's Curiosity rover were designed to facilitate gas ... 於 www.inverse.com -
#6.Access Mars by Google Creative Lab + NASA JPL
Photographed by the Curiosity Rover. Used by NASA JPL scientists. Now in your browser. Launch experiment Get the code. Collection: Web VR Experiments ... 於 experiments.withgoogle.com -
#7.Mars rover makes breathable oxygen on red planet for first time
OXYGEN ON MARS - The NASA Perseverance rover has made breathable ... on the Mars Oxygen In-Situ Resource Utilization Experiment (MOXIE), ... 於 www.imperial.ac.uk -
#8.The 5 Possibilities For Life On Mars - Forbes
... the instruments on board NASA's Mars Viking landers in 1976. There were three biology experiments performed: a gas exchange experiment, ... 於 www.forbes.com -
#9.NASA looking for people to spend a year pretending they live ...
The space agency is looking for paid volunteers to spend a year living in Mars Dune Alpha, a Martian habitat based in Johnson Space Centre in ... 於 news.sky.com -
#10.Want to Pretend to Live on Mars? For a Whole Year? Apply Now
To prepare for eventually sending astronauts to Mars, NASA began taking applications Friday for four people to live for a year in Mars Dune ... 於 www.voanews.com -
#11.NASA Rover Has Found Previously Unknown Organic ...
Using a new on-board experiment, NASA's Curiosity rover has discovered traces of previously undetected organic molecules on Mars. 於 www.sciencealert.com -
#12.Organic molecules revealed on Mars by Curiosity's new kind ...
... working with NASA's Goddard Space Flight Center has found previously unknown organic molecules on Mars using a new experiment aboard the ... 於 phys.org -
#13.MOXIE Might Be the Most Exciting Thing Perseverance Has ...
Instead, Mars Surveyor 2001 was intended to run experiments on the Martian ... Well before Mars Surveyor 2001, NASA understood that getting ... 於 spectrum.ieee.org -
#14.NASA mole failed to dig deep into Mars. What's next? - EarthSky
One key aspect of NASA's InSight mission on Mars sadly came to an end ... Seismic Experiment for Interior Structure (SEIS) – to the lander. 於 earthsky.org -
#15.NASA's Mars Exploration Program
The Mars Exploration Program studies Mars as a planetary system in order to understand the formation and early evolution of Mars as a planet, the history of ... 於 mars.nasa.gov -
#16.MARS-500 - Wikipedia
The MARS-500 mission was a psychosocial isolation experiment conducted between 2007 and 2011 by Russia, the European Space Agency, and China, in preparation ... 於 en.wikipedia.org -
#17.NASA's Perseverance rover split CO2 to make breathable air ...
It was also enough oxygen to make tiny amounts of rocket fuel. The instrument, called MOXIE (Mars Oxygen In-Situ Resource Utilization Experiment) ... 於 www.sciencenews.org -
#18.Radio Science Experiment | Instruments - NASA's Mars ...
InSight's Rotation and Interior Structure Experiment, RISE, precisely tracks the location of the lander to determine just how much Mars' North Pole wobbles ... 於 mars.nasa.gov -
#19.Indicators show potatoes can grow on Mars
This Phase Two effort of CIP's proof of concept experiment to grow ... on Mars project has been conducted by CIP with the advice of NASA ARC ... 於 cipotato.org -
#20.Nasa: The space agency are looking for volunteers to pretend ...
NASA are on the hunt to find four people who think they can live on Mars! But not on the real red planet - they want people who can pretend ... 於 www.bbc.co.uk -
#21.NASA begins year-long ′Mars isolation′ experiment - DW
NASA begins year-long 'Mars isolation' experiment. A crew testing how a small group of humans might cope with a trip to Mars has started ... 於 www.dw.com -
#22.NASA extracts breathable oxygen from thin Martian air - The ...
NASA has converted carbon dioxide from Mars' atmosphere into oxygen. Its Mars Oxygen In-Situ Resource Utilization Experiment instrument, ... 於 www.weforum.org -
#23.NASA's Perseverance rover ready for Mars landing - Los ...
If NASA's Perseverance rover lands safely on Mars, it will become the ... experiment that's crucial if humans are ever to set foot on Mars. 於 www.latimes.com -
#24.NASA looking for people to live at Mars Dune Alpha for one year
NASA plans three of these experiments, the first in fall 2022, then in 2024 and 2025. The paid volunteers will be given tasks such as simulated ... 於 www.usatoday.com -
#25.Perseverance Rover | NASA Mars Mission Facts & Video
Prepare for human exploration of Mars: the rover includes experimental technology that will attempt to produce oxygen from the Martian atmosphere. This ... 於 www.rmg.co.uk -
#26.Nasa seeks volunteers to spend a year pretending to live on ...
To simulate what it's really like to live in space, paid volunteers who take on the experiment will have very limited contact with their loved ... 於 inews.co.uk -
#27.NASA's Perseverance rover turns a tiny bit of Mars air into ...
... NASA's Jet Propulsion Lab fitted the rover with an instrument called the Mars Oxygen In-Situ Resource Utilization Experiment or MOXIE ... 於 www.engadget.com -
#28.The NASA Perseverance rover produced free oxygen on Mars
Short for Mars Oxygen In-Situ Resource Utilization Experiment, it's a toaster-size device that can convert carbon dioxide into oxygen through a ... 於 www.technologyreview.com -
#29.ESA - Mars500: study overview - European Space Agency
Crew of the Mars500 experiment ... In order to simulate a mission to Mars, six candidates (three Russian, two European and one Chinese) were sealed in an ... 於 www.esa.int -
#30.NASA is recruiting to send humans to Mars as soon as 2037
NASA is running Mars simulations where individuals will spend a month living inside 3D-printed habitats that could host the first humans on ... 於 www.wionews.com -
#31.NASA's Perseverance rover creates oxygen on Mars, for the ...
The Mars Oxygen In-Situ Resource Utilization Experiment (MOXIE) aboard the rover on Mars carried out the experiment on 20 April, which was a ... 於 theprint.in -
#32.Want to pretend to live on Mars? For a whole year? Apply now
To prepare for eventually sending astronauts to Mars, NASA began taking applications Friday for four people to live for a year in Mars Dune ... 於 apnews.com -
#33.Access Mars: A WebVR Experiment
Access Mars: Curiosity. ... The real surface of Mars. Recorded by NASA's Curiosity rover. Now in your browser. ENTER 360. try it in 360. 於 accessmars.withgoogle.com -
#34.Dynamic Albedo of Neutrons (DAN) experiment onboard ...
PDF | The description of Dynamic Albedo of Neutrons (DAN) experiment is presented, as a part of the NASA's Mars Science Laboratory mission ... 於 www.researchgate.net -
#35.Six Astronauts Spend a Year on Mars — in Hawaii - Time ...
A year in isolation on a simulated Martian plain is a good dress rehearsal for the real thing. 於 time.com -
#36.I'm Convinced We Found Evidence of Life on Mars in the 1970s
The Labeled Release experiment on the Viking mission reported positive ... experiment on NASA's spectacular Viking mission to Mars in 1976. 於 blogs.scientificamerican.com -
#37.HI-SEAS
The Hawai'i Space Exploration Analog and Simulation (HI-SEAS) is a Mars and ... (4 to 12 month) NASA Mars simulation missions and tens of other analog space ... 於 www.hi-seas.org -
#38.NASA's Perseverance Mission Begins Hunt for Ancient ...
NASA'S Ingenuity helicopter takes flight on Mars. ... With this particular experiment they only produced a small amount of oxygen. 於 news.wttw.com -
#39.NASA's Perseverance rover successfully makes oxygen on Mars
The experimental instrument developed by NASA's Jet Propulsion Laboratory is called the Mars Oxygen In-Situ Resource Utilization Experiment, or ... 於 abcnews.go.com -
#40.Can humans have babies on Mars? It may be harder than you ...
... on the surface of Mars, a team based primarily at NASA's Langley Research Center designed an experiment that would allow scientists to ... 於 www.nationalgeographic.com -
#41.Mars 2020: an essential guide to the mission - Natural History ...
In July NASA will launch a new rover headed for Mars. ... and the Radar Imager for Mars' Subsurface Experiment (RIMFAX) to peer beneath the ... 於 www.nhm.ac.uk -
#42.In first, NASA's Mars rover makes oxygen on another planet
The unprecedented extraction of oxygen, literally out of thin air on Mars, was achieved by an experimental device aboard Perseverance. 於 www.thehindu.com -
#43.NASA prepares to send astronauts to Mars by recruiting four ...
NASA is planning three of these experiments with the first one starting in the fall next year. Food will all be ready-to-eat space food and at ... 於 www.9news.com.au -
#44.NASA Wants You To Spend A Year Simulating Life On Mars ...
The agency is seeking applicants for what it calls a "one-year analog mission in a habitat to simulate life on a distant world." NASA plans to ... 於 www.npr.org -
#45.Erosion On Mars? NASA Orbiter Clicks Amazing Pics Of Red ...
NASA's Mars Reconnaissance Orbiter has gained universal recognition ... Experiment (HiRISE) camera, which clicked the fascinating images. 於 www.indiatimes.com -
#46.NASA refutes alien life found on Mars by experiment in 1970s
The experiment called the Labeled Release (LR) placed nutrients in Mars soil samples, assuming that if life were present, it would consume the ... 於 www.xinhuanet.com -
#47.With MOXIE, Perseverance will try to make oxygen on Mars
The MOXIE instrument before it was lowered into NASA's ... The golden box is called the Mars OXygen In-situ resource utilization Experiment, ... 於 www.popsci.com -
#48.NASA's Mars rover successfully touches down on the red planet
"This experiment is designed to see if we can live off the land." Perseverance will also collect samples of Martian rocks and sediments in tubes ... 於 www.nbcnews.com -
#49.What it's like to spend 8 months on a fake Mars mission - The ...
Run by the University of Hawaii, the analogue mission is funded by NASA, to learn what it takes for people to live together in a confined space ... 於 www.theverge.com -
#50.Former NASA scientist says they found life on Mars in the 1970s
Gilbert V. Levin, who was principal investigator on a NASA experiment that sent Viking landers to Mars in 1976, published an article in the ... 於 fox2now.com -
#51.NASA primed for historic flight of experimental Mars helicopter
An artist's illustration of the Ingenuity Helicopter flying on Mars. Credit: NASA/JPL-Caltech. NASA's Perseverance rover will soon release a ... 於 spaceflightnow.com -
#52.Nasa Curiosity rover has found previously undetected organic ...
“This derivatisation experiment on Mars has expanded the inventory of molecules present in Martian samples and demonstrated a powerful tool to ... 於 www.independent.co.uk -
#53.How NASA is hunting for signs of life on Mars | PBS NewsHour
In NASA's Mars mission, MOXIE means more than spunk and determination — it stands for Mars Oxygen In-Situ Resource Utilization Experiment ... 於 www.pbs.org -
#54.Explained: Why is Mars so interesting to scientists - The Indian ...
NASA's Mars 2020 Perseverance Rover touched down on the Martian surface in the early hours of Friday. The results of the experiments by ... 於 indianexpress.com -
#55.Touchdown! NASA's Mars Perseverance rover safely lands on ...
The largest, most advanced rover NASA has sent to another world touched ... The Radar Imager for Mars' Subsurface Experiment (RIMFAX) is the ... 於 www.sciencedaily.com -
#56.NASA Is Recruiting for a Mars Simulation Mission - Newsweek
NASA is seeking applicants to take part in a year-long mission that will simulate life on the surface of Mars. The mission is the first of ... 於 www.newsweek.com -
#57.Organic molecules revealed in Mars's Bagnold Dunes by ...
The wet chemistry experiments on the Sample Analysis at Mars instrument on NASA's Curiosity rover were designed to facilitate gas ... 於 www.nature.com -
#58.Science With Perseverance - NASA's Mars Exploration Program
Perseverance, NASA's first Astrobiology-focused mission, will collect and store promising samples of Mars rock and soil on the surface of the Red Planet so that ... 於 mars.nasa.gov -
#59.Curiosity Rover on Mars: Facts and Information - Space.com
NASA's Mars Rover Curiosity ... Among them is an experiment that bombards the surface with neutrons, which would slow down if they ... 於 www.space.com -
#60.NASA's Mars rover, Perseverance, aims for dicey landing to ...
18, NASA's rover Perseverance is expected to land on Mars, ... This experiment will attempt to convert carbon dioxide to oxygen. 於 www.washingtonpost.com -
#62.Nasa's InSight lander reveals internal structure of Mars - The ...
The Seismic Experiment for Interior Structure (SEIS) is a dome-shaped instrument that sits on the surface of Mars and can pick up seismic ... 於 www.theguardian.com -
#63.A NASA-funded, year-long experiment in Hawaii to mimic life ...
NASA hopes to send humans to Mars by 2030. In preparation, HI-SEAS (Hawaii Space Exploration Analog and Simulation) is running ”campaigns” made ... 於 qz.com -
#64.Scientists exit dome after yearlong Mars experiment - ABC7 ...
For the last 12 months, 6 scientists lived isolated inside of a dome in Hawaii as part of a NASA investigation into the effects of long-term ... 於 abc7news.com -
#65.NASA Is Paying People to Live in 3D-Printed Martian Habitat ...
NASA wants paid volunteers to spend a year living in a 3D-printed Martian habitat in Texas, where they will carry out spacewalks and research ... 於 www.businessinsider.com -
#66.How to prevent conflict on the way to Mars | The Economist
As the Mars crew ventures deeper into space, gaps in their communications ... Based on its HERA experiments, NASA believes it can now feed ... 於 www.economist.com -
#67.NASA's Mars Perseverance Rover Landing: How to Watch ...
MOXIE (Credit: NASA/JPL-Caltech). Perseverance is carrying technology called the Mars Oxygen In-Situ Resource Utilization Experiment (MOXIE) ... 於 www.pcmag.com -
#68.NASA is Recruiting for Yearlong Simulated Mars Mission
Each mission will consist of four crew members living and working in a 1,700-square-foot module 3D-printed by ICON, called Mars Dune Alpha. The ... 於 www.nasa.gov -
#69.3 rovers will head to Mars in 2020. Here's what you need to ...
Credit: NASA/JPL-Caltech/MSSS/JHU-APL | The Mars 2020 rover will explore ... breakdown spectrometer, oxygen experiment, Raman spectrometer 於 cen.acs.org -
#70.Mars on Earth: Apply for NASA's simulated Martian mission
It's the first of three one-year missions called Crew Health and Performance Exploration Analog, or CHAPEA, to help NASA prepare for the ... 於 www.cnn.com -
#71.NASA Rover 'Perseverance' Lands On Mars With MIT ...
NASA Rover 'Perseverance' Lands On Mars With MIT Experiment On Board ... BOSTON (CBS) – A nearly 300 million mile journey came to an end as NASA's ... 於 boston.cbslocal.com -
#72.MARIE - NASA's Mars Exploration Program
Measures particles in the range of 15 MeV to 500 MeV/nucleon. Field of View, 68 degrees. The Mars Radiation Environment Experiment (MARIE). 於 mars.nasa.gov -
#73.Mars mission: NASA's diversity Perseverance pays off - Al ...
NASA's fifth Mars rover, Perseverance, makes historic landing ... than one — its Mars Oxygen In-Situ Resource Utilization Experiment (MOXIE ... 於 www.aljazeera.com -
#74.NASA wants to pay you to live in a Mars simulation for a year
NASA is looking to recruit four people to live in a 3D-printed structure of Mars for one year, in preparation for an eventual space mission ... 於 www.cnbc.com -
#75.NASA Is Recruiting 4 People to Live in a Simulated Mars ...
During the year-long experiment, those chosen will participate in simulated space walks, scientific research, and communication exchange, as ... 於 www.travelandleisure.com -
#76.Inside the Experiment to Create Mars on Earth - Smithsonian ...
NASA is hoping to send a crew there in the 2030s, as is China, and the private company SpaceX is working to establish a permanent Martian presence with ... 於 www.smithsonianmag.com -
#77.NASA seeks applicants who want to test living in a Mars-like ...
NASA began taking applications on Friday, August 6 for an experimental project meant to prepare for eventually sending astronauts to Mars ... 於 norwaytoday.info -
#78.NASA's Curiosity Detects New Organic Molecules On Mars
The experiment has only nine cups of solvent to do the wet chemistry experiments, so they are an extremely precious commodity. Unfortunately, ... 於 www.iflscience.com -
#79.NASA's 2021 Included Mars Landing, First Flight ... - SpaceRef
Enabled more science and research to prepare humanity for missions to the Moon and Mars and benefit humans on Earth; Conducted experiments in ... 於 www.spaceref.com -
#80.NASA's Perseverance rover is different to any mission that's ...
abc.net.au/news/mars-nasa-perseverance-rover-different-to-other-missions ... will compare the results from Mars to lab experiments on Earth. 於 www.abc.net.au -
#81.Mars colony: how to make breathable air and fuel from brine
Artist's concept of astronauts and human habitats on Mars. NASA ... a name imaginatively contrived from Mars OXygen In situ Experiment. 於 theconversation.com -
#82.NASA Perseverance rover pulls breathable oxygen from air on ...
The rolling robot carries an experimental instrument about the size of a toaster called the Mars Oxygen In-Situ Resource Utilization ... 於 www.cnet.com -
#83.The Perseverance rover split CO2 on Mars to make ...
An experimental device on the NASA rover split carbon dioxide molecules into their component parts. This created enough breathable oxygen to ... 於 www.sciencenewsforstudents.org -
#84.MOXIE: Mars Oxygen ISRU Experiment - MIT Haystack ...
https://mars.nasa.gov/mars2020/spacecraft/instruments/moxie/. MOXIE, the Mars Oxygen ISRU (In-situ Resource Utilization) Experiment, has been in development ... 於 www.haystack.mit.edu -
#85.When a Mars Simulation Goes Wrong - The Atlantic
HI-SEAS is a social experiment, and the participants are the lab rats. ... NASA's Human Exploration Research Analog habitat inside a ... 於 www.theatlantic.com -
#86.NASA's MOXIE Experiment Is Making Oxygen on Mars | WIRED
Known as MOXIE, or the Mars Oxygen In-Situ Resource Utilization Experiment, the device is extracting small amounts of oxygen from the Martian ... 於 www.wired.com -
#87.Tests Indicate Which Edible Plants Could Thrive on Mars - Eos
An undergraduate experiment grew vegetables and herbs in simulated ... available Mars soil simulant similar to one developed by NASA and the ... 於 eos.org -
#88.Living the Martian Life, in Hawaii - Air & Space Magazine
A science experiment simulates what it's like to live on another planet. ... She and the others had been selected by NASA to participate in the Hawaii Space ... 於 www.airspacemag.com -
#89.Watch Video of NASA Mars Helicopter Ingenuity's First Flight
The brief test of the experimental vehicle called Ingenuity shows how explorers can study the red planet from the sky as well as the ground. 於 www.nytimes.com -
#90.NASA has produced oxygen on the surface of Mars for the first ...
The MOXIE experiment, which flew with NASA's Perseverance rover to Mars, has turned some of the planet's carbon dioxide atmosphere into ... 於 www.newscientist.com -
#91.Mars Exploration? 3 Problems Science Needs to Solve First
A year later, scientists analyzing data from NASA's Mars ... International Space Station recently conducted an experiment that showed they ... 於 now.northropgrumman.com -
#92.In a rocky Israeli crater, scientists simulate life on Mars | Reuters
A number of recent Mars probes have captivated astronomy fans across the world with robotic rovers like NASA's Perseverance and, for the first ... 於 www.reuters.com -
#93.NASA's Perseverance rover lands safely on Mars - Physics ...
The Mars 2020 mission also carried the Ingenuity Mars Helicopter, a 1.8 kg experimental device that might enable future Mars exploration to ... 於 physicsworld.com -
#94.What the NASA-funded HI-SEAS experiment taught me about ...
... to be astronauts on a mission to Mars. It was a strange time in my life, but NASA funded it: an isolation experiment called HI-SEAS on ... 於 slate.com -
#95.Oxygen has been created on Mars for the first time in space ...
The latest in NASA's multiple Mars experiments has steered the planet towards ... on the Mars Oxygen In-Situ Resource Utilization Experiment ... 於 www.euronews.com -
#96.NASA - Twitter
In 2021, our @NASAPersevere Mars rover landed and our Ingenuity helicopter took flight. Two asteroid missions launched to the skies, and another began its ... 於 twitter.com -
#97.This golden box will soon make oxygen on Mars. That's great ...
How an experiment on NASA's newest Mars rover will make oxygen to pave the way for humans on the Red Planet. 於 www.livescience.com -
#98.NASA's 2021 Included Mars Landing, First ... - PR Newswire
Providing opportunities for researchers to experiment with NASA's ECOsystem Spaceborne Thermal Radiometer Experiment on Space Station data as ... 於 www.prnewswire.com -
#99.NASA seeking American volunteers for Mars simulation
NASA is looking for eligible Americans to volunteer to assist with a Mars simulation, conducting experiments, practicing communication with ... 於 www.clickondetroit.com